The global landscape of stem cell clinical trials
Abstract
Aim: To provide a comprehensive analysis of clinical trials (CTs) listed in worldwide registries involving new applications for stem cell-based treatments and account for the role of industry. Materials & methods: We developed a data set of 4749 stem cell CTs up to 2013 in worldwide registries. We defined 1058 novel CTs (i.e., trials that were not observational in nature; did not involve an established stem cell therapy for an established indication, such as hematopoietic stem cells for leukemia; and did not investigate supportive measures). Based on trial descriptions, we manually coded these for eight additional elements. Results: Our analysis details the characteristics of novel stem cell CTs (e.g., stem cell types being tested, disease being targeted, and whether interventions were autologous or allogeneic), geotemporal trends, and private sector involvement as sponsor or collaborator. Conclusion: The field is progressing at a steady pace with emerging business models for stem cell therapeutics. However, therapeutic rhetoric must be tempered to reflect current clinical and research realities.

Databases searched were the WHO International Clinical Trials Registry Platform [103] and ClinicalTrials.gov [101]. The number of clinical trials coded into each category are in parentheses. Some clinical trials were coded for more than one category.
†See Supplementary Table 1 for full list.
‡See Supplementary Table 2 for full list.
CT: Clinical trial.

The proportion of clinical trials classified as novel applications of stem cell therapies increased from 2004 to 2011. Within novel clinical trials (n = 1058), most of the increase since 2006 was due to trials using MSCs. The decrease in clinical trials in 2012 may be due to a lag in trial registration.
MSC: Mesenchymal stem cell.

Allogeneic stem cell clinical trials increased rapidly from 2008, a few years behind autologous stem cell clinical trials.

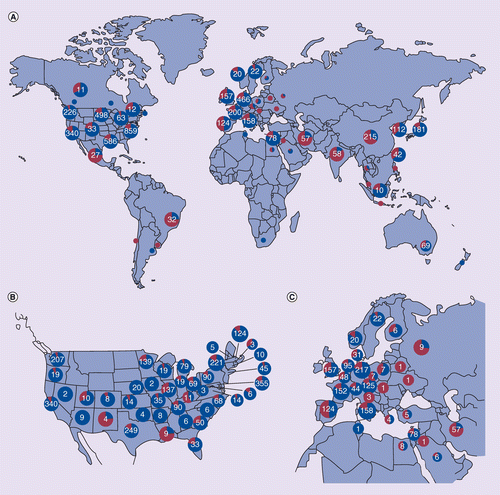
Illustrates those (A) globally, and in (B) the USA by state, and (C) Europe and the Middle East by country. The total number of trials is indicated in each blue pie chart, with the proportion of novel trials, representative of the future of regenerative medicine, indicated in red. (A) Pie charts without numbers denote regions/countries with fewer than ten clinical trials.

Stem cell therapies are a category of regenerative medicine, the promise of which includes innovative therapies for organ failure and degenerative diseases. The first human hematopoietic stem cell (HSC) transplant (HSCT) or bone marrow transplant (BMT) was performed more than 50 years ago [1], and it is estimated that more than 1 million HSCTs have been performed since [2]. While stem cell-based therapies have become the standard of care for hematological cancers and bone marrow failure states, more recently stem cell treatments have entered clinical practice for treatment of burns and corneal regeneration.
General optimism exists that stem cell-based regenerative strategies will be transformative, and there has been a rush to use stem cell products in the clinical realm [3]. This has not only led to a steady rise in stem cell clinical trials (CTs) for an expanding range of conditions [3], but also stem cell tourism in unregulated stem cell clinics around the world [4] for a wide range of illnesses [5]. Likely sources of pressure for clinical translation include: patient groups desperate for therapies and cures for currently untreatable conditions; industry and policy makers eager to see a return on substantial investments in stem cell research; and researchers, who believe that stem cell therapies will offer significant advances over current treatment or disease management options. In addition, intense media coverage has contributed to public expectations for stem cell therapies, and we have previously noted that proponents need to make a concerted effort to temper claims to reflect current research and clinical realities [3]. As stated by Daley, “premature application runs the risk of high-profile failure that would sully the credibility of this still-developing field” [6].
Beyond current therapeutic applications, novel therapies in CTs use existing products, such as HSCs, to target an expanded range of disease categories, for instance, autoimmune diseases. Alternatively, novel therapies may use new types of stem cells, including mesenchymal stem cells (MSCs), human embryonic stem cells (ESCs) or induced pluripotent stem cells (iPSCs), or cells derived from any of these cell types. MSCs are a type of stromal cell that have the potential to differentiate in vitro into a limited number of cell types, including bone, tendon, cartilage and fat, and possess immunomodulatory functions [7]. ESCs, contrarily, are pluripotent and have the ability to differentiate into every cell type in the body. In spite of much public anticipation and policy attention, ESCs have been used in a handful of CTs [3]. Currently, ESC-derived retinal pigment epithelium cells are in trials for rare retinopathies by Advanced Cell Technology (MA, USA) and partner companies (ClinicalTrials.gov identifiers: NCT01344993, NCT01469832 and NCT01345006 [101]).
In 2013, the first clinical study is commencing in Japan using iPSCs for six participants with severe age-related macular degeneration [8]. Japanese researcher, Shinya Yamanaka, received the 2012 Nobel Prize in Physiology or Medicine for the discovery of iPSCs, which are derived from mature differentiated cells reprogrammed to become pluripotent. Since the discovery in 2006, the field of iPSC research has expanded rapidly, as evident from the explosion of publications and patents in the field [9]. However, the nature of the iPSC reprogramming process raises significant safety concerns; the cells are genetically unstable in culture and have the potential for long-term tumor formation [10,11]. Efficacy will also be an issue with questions about long-term engraftment and functionality of transplanted cells [6]. Such analysis is necessary to inform regulatory policy and ethical clinical translation, a major concern internationally [12].
Beyond scientific and ethical concerns, the emerging field of stem cell therapeutics faces structural, commercial and economic challenges [13,14]. At present, most stem cell CTs remain early stage and run in academic centers. One of the greatest challenges lies in engaging industry and building multisectorial and interdisciplinary teams for large-scale efficacy trials and in the wider roll-out of successful therapies. Corporate investment decisions, the development of viable business models for the delivery of new treatments, and reimbursement decisions by public and private healthcare payers and insurers will benefit from a detailed analysis of the current types of products and services in CTs.
Accordingly, it is time to take stock of novel developments in the field, given the advancements in the knowledge of stem cell biology and the increasing clinical translational activity. Previous general reviews have discussed a subset of high-profile trials [6,15]. Other trade-related reviews focus on the regenerative medicine industry as a whole, not specifically on industry-led CTs [102]. By contrast, we have developed definitions to identify novel therapeutic applications of stem cells and for coding their characteristics. Because we have coded each CT description manually, our study accurately reflects stem cell types and uses, enabling us to identify patterns and trends for trials involving stem cells and to address industry sponsorship. This study represents the first comprehensive and systematic analysis of CTs listed in worldwide trial registries involving new applications for stem cell-based treatments. It has two aims, first to give a comprehensive account of the global landscape for stem cell therapies, and second to account for the role of industry in the field, necessary for robust development beyond its academic core.
Materials & methods
We searched the term ‘stem cell’ in ClinicalTrials.gov [101] and ‘stem cell* NOT NCT0*’ in the WHO‘s International Clinical Trials Registry Search Portal [103] to identify trials not registered with the NIH. The latter included trials from Australia and New Zealand, the UK, Brazil, China, India, South Korea, Cuba, Germany, Iran, Japan, Africa, Sri Lanka and The Netherlands. Our search for ‘stem cell’ in ClinicalTrials.gov captured more than simply that phrase. It automatically searched for related terms, including ‘blast cell’, ‘cell progenitors’, ‘cells precursor’, ‘cells stems’, ‘hematopoietic progenitor cells’, ‘hemocytoblasts’, ‘precursor cell’ and ‘progenitor cell’. However, it is important to note that registration does not imply the supervision of a competent drug regulatory authority. While many of the trials we analyzed were likely registered with an agency such as the US FDA, many were not. For example, research listed for trials in Japan may be registered with the Ministry of Health but not the Pharmaceuticals and Medical Devices Agency, the Japanese equivalent of the FDA, and therefore belong to a separate category of clinical research in the Japanese system. The Biomaster (Japan) and Cytori Therapeutics, Inc. (Japan) industry trials are examples of ministry-registered trials.
Our search strategy had some limitations. Some CTs involving stem cells did not explicitly include ‘stem cell’ or related terms in the trial registration entry. For example, Geron Corporation’s high-profile CT using ESCs for spinal cord injury does not mention ‘stem cell’ or related terms once in its ClinicalTrials.gov registry entry (ClinicalTrials.gov identifier: NCT01217008) [101]. Thus, some stem cell trials were not captured by our search. In addition, our focus was on stem cell CTs, not all CTs that may be categorized as regenerative medicine. For example, engineered tissue products, such as the Apligraf® skin graft by Organogenesis (MA, USA), were not included. Apligraf is cultured from fibroblasts and keratinocytes. However, a comparison of our data set to a broader data set of cell therapies compiled by the research group of Chris Mason and Emily Culme-Seymour at the London Regenerative Medicine Network (UK) showed good concordance in our two CT data sets for all complete years up to the end of 2009 [Culme-Seymour E, Pers. Comm.]. The London group selected cell therapy CTs from a larger set (n = 17,362 to mid-2010) compiled by searching ClinicalTrials.gov in mid-2010 using the terms ‘cell’ and ‘therapy’. After filtering for relevance (actual cell therapy compared with an enabling technology), the London data set included 2584 trials, of which 1765 also occurred in our data set (2804 CTs to 2009 from ClinicalTrials.gov [101]).
We removed nonstem cell-related trials that were nevertheless captured by the search and duplicates (trials registered in more than one database) and constructed a data set of CT information using all fields from ClinicalTrials.gov whenever possible. We limited the data set to CTs registered in either registry before 1 January 2013.
▪ Definition of novel CT
We established criteria to identify those CTs testing novel stem cell therapies. These novel applications of stem cell therapies involved:
▪ The use of stem or progenitor cells to stimulate nonhematopoietic organ regeneration (e.g., limbal stem cells for cornea regeneration or HSCs for cardiac repair);
▪ The use of agents to stimulate stem or progenitor cell action for regenerative or therapeutic purposes (note that CTs on agents targeting cancer stem cells were excluded);
▪ The use of established HSCT procedures for novel indications (i.e., HSCT for autoimmune or congenital diseases);
▪ The use of novel agents or processes for stem or progenitor cell mobilization for therapeutic purposes;
▪ The use of gene therapy or other ex vivo modified stem or progenitor cells (note that adoptive cell therapies for targeting cancers were not classified as novel).
We excluded CTs that:
▪ Were observational in nature (e.g., only measuring circulating endothelial precursor cells after exercise);
▪ Involved an established stem cell therapy for an established indication (e.g., HSCT for leukemia); or
▪ Investigated supportive measures surrounding a stem cell therapy (e.g., antibiotics to prevent infection in HSCT recipients).
▪ CT coding
We established a data set that captured all available fields from the originating database for all CTs included in the data set. The data set included date, ID number, title, abstract, sponsor, location and phase. For novel CTs, based on the title and the abstract, each trial was coded for eight elements (Figure 1):
▪ The goal of the therapy;
▪ The target of the therapy;
▪ The mechanism or process leading to the disease being treated;
▪ The disease/condition targeted;
▪ The stem cell tissue source;
▪ Any stem cell manipulation;
▪ The graft donor source;
▪ Stem cell type.
Wherever possible, we categorized the stem cells used. Three coders were trained by H Atkins to code the data set. One (MD Li) coded all trials and two worked during different time periods, prior to 2011 and post 2011. Where discrepancies and questions occurred, these were resolved either through discussion among the coders and/or verified through discussions with H Atkins. If further information was required for accurate coding, we conducted a keyword Google™ search (e.g., to determine the identity or characteristics of a therapeutic agent identified only by proprietary title in the registry entry). Industry trials were those that, in the registry data field for sponsor and collaborator, indicated a company name. We then searched for each company name in the Orbis – Bureau van Dijk database for financial data, if available, in September 2013 [104].
Results
▪ The characteristics of novel CTs
Our search yielded 4749 CTs registered before 1 January 2013. Based on our definition (see ‘Materials & methods’ section), we classified 1058 (22%) as novel applications of stem cell therapy (Figure 1; see also Supplementary Material for detailed descriptions of the characteristics of the 1058 novel CTs). The proportion of novel CTs increased from 2004 to 2011 (Figure 2). The bulk of the increase has occurred since 2006 due to CTs using MSCs. The majority (87%) of novel CTs were in early-phase testing (Supplementary Figure 1). Novel CTs most commonly had a regenerative therapeutic goal (87%), and this subset of CTs primarily targeted tissue injury from degeneration and ischemia (Figure 1).
The majority of stem cell products used were obtained from a hematopoietic source such as the bone marrow (41%), peripheral blood (16%) or umbilical cord (9%). An increasing number of trials tested stem cell products derived from adipose tissue (9%; Supplementary Table 1). Forty-two percent of CTs used products produced by in vitro cell culture, mainly MSCs. Other trials used stem cell products that had been purified (22%), manipulated with drug treatments (7%) or genetically modified (7%; Figure 1). Since 2004, there has been a steady number (7–15 per year) of CTs using interventions such as drug treatments and exercise for in vivo mobilization or activation of stem cells, usually without stem cell harvest or treatment. Notably, the use of allogeneic products has increased rapidly since 2009, although autologous uses are still more numerous (Figure 3).
▪ Diseases or conditions addressed by novel stem cell CTs
Unlike the majority of CTs that primarily addressed hematological and other cancers, the focus of novel stem cell CTs, with respect to disease indication, is cardiovascular disease (Figure 1). Nevertheless, the immune system remains the most common target of stem cell therapies for various disease indications, which include cancers, graft-versus-host disease (GvHD) and other nonmalignant hematologic conditions, as well as autoimmune diseases such as multiple sclerosis, Crohn‘s disease and Type 1 diabetes. Increasing numbers of CTs targeted neurological diseases, while CTs for liver disease, bone and cartilage conditions, diabetes, and eye diseases remained rare (<70 CTs globally; Figure 1). These diseases and conditions were reflected in the tissues targeted by the CTs (Supplementary Figure 2 & Supplementary Table 2). The Supplementary Material presents detailed summaries of the CTs in each disease category, including the extent of industry engagement within each disease category.
Development of cardiovascular indications were in the most advanced stage of testing, with 24% of CTs beyond Phase II, compared with just 13% of all CTs.
▪ International landscape of novel stem cell CTs
The registration and initiation of novel CTs has expanded rapidly since 2004 (Figure 4). In 2008, the number of novel trials in Asia, mainly in China, but also in South Korea, India and Japan, surpassed the number in the USA and Europe. This geographic shift continues to become more pronounced. An increasing number of trials are taking place in Australia, Brazil, India, South Korea, Iran and Israel (Figure 5). Countries in Asia, the Middle East and South America host a greater proportion of novel CTs (Figure 5A). In the USA, states with policies and funding to support stem cell research also dominate CTs. States with more than 100 total CTs include Maryland (mainly NIH CTs), California, Texas, New York, Washington, Minnesota, Illinois and Massachusetts. Maryland, Illinois, California and Texas have the most novel CTs (>30 CTs; Figure 5B). In Europe, the dominant countries for novel stem cell CTs are Spain, the UK, and Germany, but there are also many in Italy, France, The Netherlands, and Belgium (Figure 5C).
▪ Industry engagement in novel stem cell CTs
Industry involvement was reported in 265 (25%) novel CTs, either as principal sponsor or collaborator. This does not include Clinical Research Organizations (CROs) responsible for running many trials. The extent of industry involvement has grown rapidly since 2004 (Figure 6), and our study likely underestimated its extent; we only captured sponsorships or collaborations that were reported in the CT databases. Internationally, commercial sponsors were involved in more than 25% of novel CTs in South Korea, Malaysia, Canada, Israel, India, Australia and the USA (all countries with >5 CTs; Figure 5). The USA had the greatest absolute number of CTs with industry involvement. The US states reporting the most industry involvement as a proportion of novel CTs were Louisiana, California, North Carolina, Pennsylvania and Massachusetts (all states with >5 CTs; Figure 5B). Indeed, in California, nearly 50% of novel CTs had industry involvement. However, most CTs globally with industry involvement were in an early (Phase I, I/II and II) stage (Supplementary Figure 2). As a proportion of novel CTs, industry was most heavily engaged in gastrointestinal diseases (48%), lung disease (40%), cartilage disease (36%), neurological diseases (28%), diabetes (26%) and bone conditions (25%).
The majority of companies involved were small-to-medium-sized, privately held biotechnology companies with fewer than 80 employees engaged in one or two CTs (Supplementary Table 3). Some companies focused on one or two diseases, including Advanced Cell Technology and Mesoblast (Australia), while others limited their trials to a discipline such as cardiovascular diseases (e.g., Capricor [CA, USA] and Cardio3 BioSciences [Belgium]), stroke (e.g., ReNeuron [UK], Stem Cell Therapeutics [ON, Canada] and Sanbio [CA, USA]), and cartilage or bone repair (e.g., Mesoblast, NuVasive® [CA, USA] and Orthofix [TA, USA]). An emerging business model, linked to the rise in MSC CTs, is the allogeneic use of a single stem cell product for a broad range of conditions (Supplementary Table 3). Some regenerative medicine biotechnology firms, such as Athersys (OH, USA), Osiris (MD, USA) and Medipost (South Korea) were engaged in trials for multiple, unrelated conditions. Smaller biotechnology companies have engaged the largest players in the pharmaceutical industry in the joint ventures to produce allogeneic stem cell products (e.g., Gamida Cell [Israel]–Teva [Israel] joint venture using StemEx® for hematological malignancies and Athersys–Pfizer (NY, USA) using MultiStem® for ulcerative colitis) as the latter are focused on product development for broad-based use. Within this paradigm, more traditional pharmaceutical companies’ (e.g., Pfizer, Takeda [Japan] and Italia Farmaceutici [Italy])activity in the stem cell field remains focused upon biological and small molecules for stem cell mobilization and/or activation (Supplementary Table 3). Novartis Pharmaceuticals (NJ, USA) stands out in conducting a trial using ex vivo-expanded umbilical cord blood stem cells for hematological malignancies (see the Supplementary Material for detailed summaries of the industry trends in each disease category).
Discussion
▪ Current state of translational stem cell research
Our results show that the majority of all registered stem cell CTs continue to address uses of HSCs derived from bone marrow, umbilical cord blood or peripheral blood, for conditions traditionally associated with BMT. However, even in the well-established HSC field, a small but growing number of trials are for novel indications, such as multiple sclerosis, Type 1 diabetes and other autoimmune diseases.
There is increasing activity in clinical testing of MSCs derived from bone marrow or adipose tissue. Major gaps in knowledge exist in the fundamental understanding of: the duration of their survival after transplantation; their impact on unaffected tissues and organs; and the phenotypic changes they undergo in the target tissue. Daley has raised concerns over this expansion of MSC trials into “indications where the clinical hypotheses are more speculative, the therapeutic mechanisms are incompletely defined, and in some instances the preclinical evidence is highly contentious” [6]. Despite these gaps, MSCs are being tested around the globe for a wide range of conditions. Indeed, in our analysis, MSCs were the predominant stem cell used in novel clinical applications. Outside of North America and Europe, MSCs were used in an even higher proportion of novel trials of stem cells. We have previously speculated that North America and Europe are dominant in trials extending the traditional use of HSCs because of the existing BMT infrastructure. By contrast, this infrastructure is underdeveloped in many regions that are conducting proportionately more novel stem cell CTs using MSCs [3,16].
Some trials of MSCs are being conducted to examine their regenerative or reparative potential including Phase II trials for diabetes, pulmonary hypertension and chronic obstructive pulmonary disease (COPD) [17]. However, 126 CTs involving MSCs were attempting to exploit their immunomodulatory properties, most commonly for treating GvHD. The underlying mechanism for these properties is not fully understood but is based on observations that MSCs interfere with immunological assays and modulate classes of immune cells [15]. This observation has led to MSC CTs for autoimmune diseases such as Crohn’s disease [18], multiple sclerosis and systemic lupus erythematosus, as well as diabetes [19]. Efficacy outcomes are difficult to interpret because of variable pre-MSC transplant treatment regimens, nonstandardized outcome measures and lack of long-term follow-up [18,20]. However, allogeneic-cultured MSCs produced under proprietary conditions and marketed as Prochymal® have received a notice of compliance with conditions in Canada for the treatment of pediatric steroid-refractory GvHD, which represents the first stem cell biologic licensed by a national regulatory agency [105]. Prochymal is being evaluated in Phase III CTs for GvHD and Crohn’s disease, and is the only stem cell product currently designated by the FDA as both an orphan drug and a fast-track product [106]. Furthermore, Phase II trials are ongoing in chronic obstructive pulmonary disease, Crohn’s disease, GvHD, myocardial infarction and Type 1 diabetes.
Cardiovascular diseases are the leading condition for registration of novel stem cell CTs. These mainly attempted to regenerate the heart or peripheral vascular system through various stem cell infusions or mobilization of endogenous stem cells. Regarding trials addressing cardiac conditions in particular, overall safety appears generally favorable [21,22]. However, the limited clinical data suggest that few donor cells remain in the heart following transplantation [22]. Only modest improvements in cardiac function after myocardial infarction have been reported using a range of cells, including MSCs, skeletal myoblasts, bone marrow cells, peripheral blood cells [23] or autologous cardiac stem cells derived from heart biopsies with no clear choice of optimal cell type emerging [22]. A recent update of the 2008 Cochrane Review of 33 trials addressed whether bone marrow-derived stem cells could prevent damage caused by a heart attack. It concluded that there is moderate long-term improvement of heart function and reduction of scar size, but lack of sufficient evidence to evaluate long-term survival rates [24], although this may not be a relevant end point when the cell therapy is not directed at reversal of atherosclerosis or infarct prevention.
Criticism has arisen from the perceived lack of rigor and absence of controlled trials. Increased rigor requires the involvement of homogeneous patient populations, and consistency in cell phenotyping practices, dosing, delivery and frequency of application [22,23,25]. The task force of the European Society of Cardiology for Stem Cells and Cardiac Repair recently received funding from the EU to conduct a large-scale trial with 3000 patients using standardized treatment procedures [24]. It is expected that, as the field matures, there will be efforts to increase harmonization among international trial sites and to conduct larger double-blind, placebo-controlled studies. Such efforts will be required to convince patients, the medical community and regulators of the efficacy of stem cell therapies, and lead to increased industry participation and investment in the field, and ultimately to convince reimbursement agencies that such therapies are cost effective relative to current available and other new competing treatments.
Similar concerns have been expressed for stem cell treatments of diseases of other organs, although research efforts are focused at the beginning of the path towards clinical application. The pressure for rapid testing and implementation are most pronounced for neurological conditions because, for many diseases, there is a lack of treatment options [3]. Many trials are still in very early stages and run in academic centers [26]. Progress is hampered by the lack of funding streams beyond the public sector [27]. Optimistically, overall, 28% of novel neurological CTs indicated industry involvement, which increases to nearly 50% in the USA, and over 50% in India and South Korea. On the other hand, StemCells Inc. (CA, USA) discontinued its trial for a rare neurological condition, Batten’s disease, because of problems with recruitment [6]; the company’s current focus is in Pelizaeus–Merzbacher disease, a rare genetic leukodystrophy. The highly publicized Geron (CA, USA) trial using oligodendrocyte progenitors derived from human ESCs for remyelination of damaged axons following spinal cord injury was first delayed by the FDA over concerns of cyst formation in animal models and was then halted in November 2011, after only four trial subjects were treated, when Geron discontinued its stem cell program [28]. We agree with Daley’s assessment that, while there are promising advances, routine clinical use of stem cell therapy for neurological conditions is still optimistically many decades away [6].
Clinical use of pluripotent stem cells has shifted to ophthalmic indications. Advanced Cell Technology has reported on the safety of retinal pigment epithelium cells derived from ESCs transplanted into patients with advanced-stage Stargardt’s macular dystrophy and dry age-related macular degeneration [29]. Similarly, ESCs can be cultured to ‘corneal-like cells’ in vitro[30,31]. Adult limbal stem cells maintain and regenerate the corneal epithelium, and while limbal stem cell transplantation is currently used to regenerate damaged corneas in certain situations, a source of limbal stem cells is not always available. ESC-derived limbal stem cells may provide an alternative source. Finally, advances in directed differentiation of pluripotent stem cells into retinal cell lineages, combined with iPSC production and research on in vitro creation of 3D retinas, offers the promise of vision restoration to patients with retinal diseases [32].
▪ Beyond the academy: industry engagement in stem cell CTs
Our analysis of industry-sponsored CTs indicates that a few potential business models are emerging for stem cell therapies. Private and public biobanks have been established to store and supply umbilical cord blood-derived stem cells mainly for pediatric HSCT. Stem cell biobanks have been set up and networked in a variety of regions to enhance access to ESC and iPSC lines. However, most still only supply research-grade materials.
The point-of-care device business model, which builds on traditional applications of HSC transplantation where a stem cell laboratory is located in academic centers or hospitals, is based on companies that produce devices that manipulate the cells on site for immediate use. An example is Cytori Therapeutics‘ Celution® device, which “automates and standardizes the extraction, washing and concentration of autologous adipose-derived cells, which can then be reimplanted back into the same patient in a single surgical procedure” [13]. Where cells are processed off site or where more extensive manipulation is involved, access to facilities meeting GMP standards and clinical grade materials will be limiting [33]. The National Heart Lung and Blood Institute of the NIH is remedying the gap in appropriate manufacturing facilities by supporting Production Assistance for Cellular Therapies (PACT) and its five current GMP facilities in the USA. PACT’s purpose is to provide product development and manufacturing assistance to researchers who require current GMP facilities but lack such capacity [34]. PACT also assists in early-stage CTs and provides technology transfer support for clinical-scale production.
Biotechnology companies are also engaged in the development of cell therapies that allow for an ‘off-the-shelf’ approach [14,33]. This business model is commercially attractive, as a common cell product could be used to treat large patient populations, generating a steady revenue stream. In this approach, therapies are standardized, allowing for scaled manufacturing and bulk production. However, the allogeneic nature of these products introduces immunological issues that will certainly hinder the adoption of this model [35]. In light of this concern, it is not surprising that 63% of novel allogeneic CTs involved MSCs, which have immunosuppressive properties. Similar off-the-shelf approaches are being used by traditional pharmaceutical and biotechnology companies engaged in the development of drugs that act on stem cells. These small-molecule drugs target stem cells, causing them to mobilize and/or differentiate in vivo. Although this model allows for scalability and the creation of blockbuster drugs with high margins, few firms are as yet actively engaged in the field [33].
Our study likely under-represented industry sponsorship of CTs because it is reliant solely on global CT registries and did not canvass trade sources. Registries have limitations in terms of scope and language of coverage, and the reporting of international trials is based on voluntary and specific registration. Furthermore, registration is not indicative of supervision by a competent drug regulatory authority. Although beyond the scope of this analysis, it is important to note that companies engaged in providing unproven stem cell therapies in clinics linked to ‘stem cell tourism’ register CTs as a marketing tactic or as a method to recruit patients. Such registration adds a veneer of legitimacy to the CT and companies, such as Adistem (China), Beike Bio-technology (China) and subsidiaries TCA Cellular Therapy (LA, USA), RNL Bio (South Korea) and Bioheart (FL, USA), have all registered CTs (Supplementary Table 3). Registries might be improved by indicating the competent regulatory authority and research ethics board overseeing the trial.
Conclusion & future perspective
Our study presents the most comprehensive account of the global stem cell CT landscape to date. It is evident from our analysis that the breadth and number of CTs is increasing around the globe, but that the whole endeavor is proceeding with caution. The large number of Phase I trials demonstrates that the field is just starting to move from preclinical research into the clinic. The focus has largely been on the safety of these treatments; however, consensus is emerging that large-scale safety concerns have not arisen, at least in the short term for many cultured stem cell types. The safety of pluripotent stem cells is still an unknown quantity given that they are relatively unstable in culture and there has been limited exposure in human trials. Therefore, clinical translation should proceed with caution and with sufficient regulatory oversight.
Future research should expand our approach to other categories of regenerative medicine, such as cell therapies, immunotherapy and tissue engineering; give a more comprehensive account of industry CTs by searching websites and press releases of regenerative medicine companies and trade publications; and identify and analyze published CT results to more accurately account for progress in the field. There is, as of now, limited evidence of efficacy for novel stem cell therapeutic applications. It is unclear whether these preliminary results from current CTs will lead to more public or private investment that will spearhead definitive multicenter CTs that demonstrate the regenerative potential of stem cells trials or whether it will be back to the bench. The field is progressing at a steady pace, but the therapeutic rhetoric must be tempered to reflect current clinical and research realities.
Executive summary
Rationale & aims
▪ Stem cell-based regenerative medicine strategies are expected to be transformative for organ failure and degenerative diseases. As translational activity increases, it is time to take stock of novel and global developments in the field of translational stem cell research.
▪ This analysis aims to give a comprehensive account of the global landscape for stem cell therapies and to account for the role of industry in the field, necessary for robust development beyond its academic core.
Materials & methods
▪ We developed a data set from a search of the term ‘stem cell’ (and synonyms) in ClinicalTrials.gov and ‘stem cell* NOT NCT0*’ in the WHO‘s International Clinical Trials Registry Search Portal, and coded these according to criteria we developed to identify clinical trials (CTs) testing novel stem cell therapies.
Characteristics of novel stem cell CTs
▪ Since 2006, an increasing number and proportion of novel CTs have tested mesenchymal stem cells derived from bone marrow or adipose tissue. A growing number of trials use hematopoietic stem cells for novel indications, such as autoimmune diseases.
▪ Cardiovascular diseases are the main target of novel CTs.
▪ Most novel trials remain focused on safety, and there is as yet limited evidence of efficacy for many indications.
International landscape
▪ The registration of novel stem cell CTs is expanding rapidly around the world, particularly in east Asia, but also in Australia, Brazil, India, Iran and Israel.
Industry involvement
▪ Industry involvement has grown rapidly since 2004 and was reported in a quarter of novel CTs, either as principal sponsor or collaborator. This is likely an underestimate because we only captured sponsorships or collaborations that were reported in the CT databases. Industry was, proportionally, most heavily engaged in gastrointestinal diseases (48%), lung disease (40%), cartilage disease (36%), neurological diseases (28%), diabetes (26%) and bone conditions (25%).
▪ A few potential business models are emerging for stem cell therapies, notably ‘point-of-care devices’ and ‘off-the-shelf’ models.
▪ The majority of companies involved were small to medium-sized, privately held biotechnology companies.
Conclusion
▪ The field is progressing at a steady pace, but the therapeutic rhetoric must be tempered to reflect current clinical and research realities. It is unclear whether results from current trials will lead to more public or private investment that will spearhead definitive multicenter CTs that demonstrate the regenerative potential of stem cells trials or whether it will be back to the bench.
Future perspective
▪ Future research should expand our approach to other categories of regenerative medicine, such as cell therapies, immunotherapy and tissue engineering; give a more comprehensive account of industry CTs by searching websites and press releases of regenerative medicine companies and trade publications; and identify and analyze published CT results to more accurately account for progress in the field.
Author contributions
T Bubela and H Atkins designed the research questions; MD Li and H Atkins developed the final clinical trial coding frame and completed the data analysis; and MD Li, H Atkins and T Bubela drafted the manuscript and approved the final version for publication.
Acknowledgements
The authors would like to thank M Hafez for development of the initial coding frame and coding clinical trials to 2009, M Bieber for technical assistance with mapping and data management, and L Dacks and M Fung for research assistance.
Financial & competing interests disclosure
This study was funded by the Stem Cell Network Strategic Core Grant (to T Bubela and H Atkins) and a Stem Cell Network Strategic Core Grant: Public Policy & ELSI (to T Bubela and H Atkins). In 2011, MD Li was awarded an Alberta Innovates Health Solutions Summer Studentship and a Stem Cell Network Social Sciences Summer Studentship. H Atkins is a stem cell transplant physician at the Ottawa Hospital Research Institute and is currently the principal investigator of a clinical trial using hematopoietic stem cell transplantation for multiple sclerosis. The authors have no other relevant affiliations or financial involvement with any organization or entity with a financial interest in or financial conflict with the subject matter or materials discussed in the manuscript apart from those disclosed.
No writing assistance was utilized in the production of this manuscript.
Open Access
This work is licensed under the Creative Commons Attribution-NonCommercial 3.0 Unported License. To view a copy of this license, visit http://creativecommons.org/licenses/by-nc-nd/3.0/
Papers of special note have been highlighted as:▪ of interest ▪▪ of considerable interest
References
- 1 Appelbaum FR. Hematopoietic-cell transplantation at 50. N. Engl. J. Med.357(15),1472–1475 (2007).
- 2 Thomas ED, Lochte HL Jr, Lu WC, Ferrebee JW. Intravenous infusion of bone marrow in patients receiving radiation and chemotherapy. N. Engl. J. Med.257(11),491–496 (1957).
- 3 Bubela T, Li MD, Hafez M, Bieber M, Atkins H. Is belief larger than fact: expectations, optimism and reality for translational stem cell research. BMC Med.10,133 (2012).
- 4 Regenberg AC, Hutchinson LA, Schanker B, Mathews DJ. Medicine on the fringe: stem cell-based interventions in advance of evidence. Stem Cells27(9),2312–2319 (2009).
- 5 Lau D, Ogbogu U, Taylor B, Stafinski T, Menon D, Caulfield T. Stem cell clinics online: the direct-to-consumer portrayal of stem cell medicine. Cell Stem Cell3(6),591–594 (2008).▪▪ Key empirical study on stem cell tourism clinics, the conditions for which they market therapies and the evidence base for those therapies.
- 6 Daley GQ. The promise and perils of stem cell therapeutics. Cell Stem Cell10(6),740–749 (2012).▪▪ Review of the clinical trial environment for stem cell therapies. Comments on the difficulties in obtaining unequivocal evidence for robust clinical benefit, the rise of stem cell clinics, and the challenges and technical barriers for achieving meaningful clinical impact.
- 7 Dominici M, Le Blanc K, Mueller I et al. Minimal criteria for defining multipotent mesenchymal stromal cells. The International Society for Cellular Therapy position statement. Cytotherapy8(4),315–317 (2006).
- 8 Cyranoski D. Stem cells cruise to clinic. Nature494(7438),413 (2013).
- 9 Mathews DJ, Cook-Deegan R, Bubela T. Patents and misplaced angst: lessons for translational stem cell research from genomics. Cell Stem Cell12(5),508–512 (2013).
- 10 Pera MF. The dark side of induced pluripotency. Nature471(7336),46–47 (2011).
- 11 Zwaka TP. Stem cells: troublesome memories. Nature467(7313),280–281 (2010).
- 12 Hyun I, Lindvall O, Ahrlund-Richter L et al. New ISSCR guidelines underscore major principles for responsible translational stem cell research. Cell Stem Cell3(6),607–609 (2008).▪▪ Key statement of principles from the International Society for Stem Cell Research on the responsible translation of stem cell research.
- 13 Mason C, Brindley DA, Culme-Seymour EJ, Davie NL. Cell therapy industry: billion dollar global business with unlimited potential. Regen. Med.6(3),265–272 (2011).
- 14 Rao MS. Funding translational work in cell-based therapy. Cell Stem Cell9(1),7–10 (2011).
- 15 Trounson A, Thakar RG, Lomax G, Gibbons D. Clinical trials for stem cell therapies. BMC Med.9,52 (2011).▪ Selective review of key clinical trials for stem cell therapies using a range of stem cell types for a range of conditions.
- 16 Gratwohl A, Baldomero H, Frauendorfer K et al. Hematopoietic stem cell transplantation: a global perspective. JAMA303(16),1617–1624 (2010).
- 17 Weiss DJ, Bersoncello I, Borok Z, Kim C et al. Stem cells and cell therapies in lung biology and lung diseases. Proc. Am. Thorac. Soc.8(3),223–272 (2011).
- 18 Dalal J, Gandy K, Domen J. Role of mesenchymal stem cell therapy in Crohn’s disease. Pediatr. Res.71(4 Pt 2),445–451 (2012).
- 19 Barcala Tabarrozzi AE, Castro CN, Dewey RA, Sogayar MC, Labriola L, Perone MJ. Cell-based interventions to halt autoimmunity in Type 1 diabetes mellitus. Clin. Exp. Immunol.171(2),135–146 (2013).
- 20 Tyndall A. Application of autologous stem cell transplantation in various adult and pediatric rheumatic diseases. Pediatr. Res.71(4 Pt 2),433–438 (2012).
- 21 Williams AR, Hare JM. Mesenchymal stem cells: biology, pathophysiology, translational findings, and therapeutic implications for cardiac disease. Circ. Res.109(8),923–940 (2011).
- 22 Rosenweig A. Cardiac regeneration. Science338(6114),1549–1550 (2012).
- 23 Ptaszek LM, Mansour M, Ruskin JN, Chien KR. Towards regenerative therapy for cardiac disease. Lancet379(9819),933–942 (2012).
- 24 Mummery CL, Lee RT. Is heart regeneration on the right track? Nat. Med.19(4),412–413 (2013).
- 25 Lawall H, Bramlage P, Amann B. Treatment of peripheral arterial disease using stem and progenitor cell therapy. J. Vasc. Surg.53(2),445–453 (2011).
- 26 Bednar MM, Perry A. Neurorestoration therapeutics for neurodegenerative and psychiatric disease. Neurol. Res.34(2),129–142 (2012).
- 27 Aboody K, Capela A, Niazi N, Stern JH, Temple S. Translating stem cell studies to the clinic for cns repair: current state of the art and the need for a Rosetta stone. Neuron70(4),597–613 (2011).
- 28 Baker M. Stem-cell pioneer bows out. Nature479(7274),459 (2011).
- 29 Schwartz SD, Hubschman JP, Heilwell G et al. Embryonic stem cell trials for macular degeneration: a preliminary report. Lancet379(9817),713–720 (2012).
- 30 Ahmad S. Concise review: limbal stem cell deficiency, dysfunction, and distress. Stem Cells Transl. Med.1(2),110–115 (2012).
- 31 Osei-Bempong C, Figueiredo FC, Lako M. The limbal epithelium of the eye – a review of limbal stem cell biology, disease and treatment. BioEssays35(3),211–219 (2013).
- 32 Tibbetts MD, Samuel MA, Chang TS, Ho AC. Stem cell therapy for retinal disease. Curr. Opin. Ophthalmol.23(3),226–234 (2012).
- 33 Martin PA, Coveney C, Kraft A, Brown N, Bath P. Commercial development of stem cell technology: lessons from the past, strategies for the future. Regen. Med.1(6),801–807 (2006).▪ Key article on the challenges facing the commercial development of stem cell technologies that builds upon a large empirical study of the sector.
- 34 Reed W, Noga SJ, Gee AP et al. Production Assistance for Cellular Therapies (PACT): four-year experience from the United States National Heart, Lung, and Blood Institute (NHLBI) contract research program in cell and tissue therapies. Transfusion49(4),786–796 (2009).
- 35 von Tigerstom B. The Food and Drug Administration, regenerative sciences, and the regulation of autologous stem cell therapies. Food Drug Law J.66(4),479–506 (2011).
- 101 NIH. ClinicalTrials.gov. http://clinicaltrials.gov (Accessed 12 October 2013)
- 102 Alliance for Regenerative Medicine. ARM 2013 Regenerative Medicine Annual Report. http://alliancerm.org/news/download-must-have-industry-report (Accessed 12 October 2013)
- 103 WHO. International Clinical Trials Registry Platform. http://apps.who.int/trialsearch (Accessed 12 October 2013)
- 104 Bureau Van Dijk. Orbis. www.bvdinfo.com (Accessed 12 October 2013)
- 105 Rattue P. Prochymal – First Stem Cell Drug Approved. Medical News Today (2012). www.medicalnewstoday.com/articles/245704.php (Accessed 12 October 2013)
- 106 Osiris Therapeutics, I (2013). Therapeutics. Prochymal. www.osiris.com/therapeutics.php (Accessed 12 October 2013).