Abstract
Dengue is the most important mosquito-borne viral pathogen globally, with approximately 100 million cases of acute dengue annually. Infection can result in severe, life-threatening disease. Currently, there is no effective vaccine or licensed antiviral. Management is primarily supportive with fluids. Direct antiviral therapies that reduce dengue severity could be useful although these would need to inhibit all four viral serotypes effectively. This review focuses on the interventions that currently considered the gold standard in case management as well as exploratory therapies that have been studied in clinical trials. Although antiviral drug and therapeutic antibodies for dengue remain a work in progress, these studies have produced some promising results and may have the potential to be future drugs.
First draft submitted: 20 April 2015; Accepted: 25 August 2015; Online: 23 November 2015
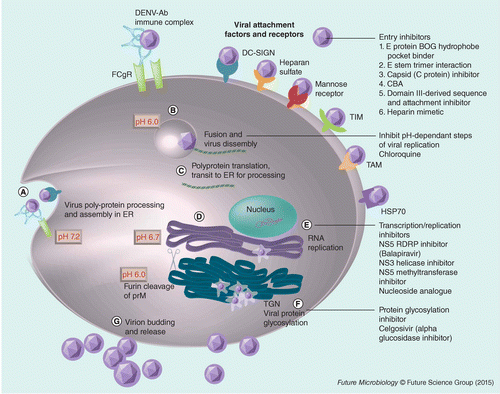
(A) Virion binds to receptors on plasma membrane and enters the cell via receptor-mediated or Fc-receptor-mediated phagocytosis if DENV is opsonized to cross-reactive or subneutralizing levels of antibodies. (B) Within the acidic endosomal compartment, the E protein undergoes conformational change to mediate fusion of the viral membrane with the endosome membrane, releasing the positive-stranded genomic RNA into the cytoplasm. (C) Genomic RNA is translated into polyprotein. (D) Polyprotein is cleaved into individual proteins on the endoplasmic reticulum (ER) membranes by viral and cellular proteases. (E) RNA replication occurs on the ER membranes in association with the viral replication complex composed of NS1, NS2A, NS2B, NS3, NS4A and NS5. Viral assembly produces immature viral particles. (F) Immature viral particles (shown as spiky virus particles) are transported through the secretory pathway. Lower pH in the trans-Golgi network activates the host protease, furin to produce mature DENV particles (shown as smooth virus particles). (G) Release of mature virus into the extracellular space. Boxes represent target sites of antiviral drugs or compounds. The bold red numbers refer to the pH values of the respective compartments.
Dengue virus (DENV) is the most prevalent mosquito-borne viral hemorrhagic fever. DENV is entrenched in over 100 countries in the tropics [1]. Endemic virus transmission, coupled with frequent cyclical epidemics, puts almost half of the world’s population at risk of infection each year [2–4]. Both DENV and its mosquito vectors, principally Aedes aegypti, are also encroaching into nonendemic areas due to increasing international travel and rapid urbanization with poor public health infrastructure. Recent trends in global warming could further exacerbate the spread of dengue vectors both northward and southward [5,6]. Besides causing mortality, the cost of acute illness to society is considerably resulting from loss of productivity to costs arising from medical care [7,8].
DENV belongs to the Flaviviridae family. There are four antigenically distinct serotypes of this virus (DENV-1, 2, 3 and 4) [9]. A fifth serotype has been suggested although data demonstrating its antigenic distinctness from the other four DENVs remain to be reported in the literature [10]. The virus is enveloped and possesses a single-stranded positive-sense RNA genome [11]. The genome is translated as a single polyprotein, which is then cleaved by host and viral proteases into three structural proteins that collectively package the RNA genome: capsid (C), premembrane (prM), membrane (M) and envelope (E) and seven nonstructural proteins (NS1, NS2A, NS2B, NS3, NS4A, NS4B and NS5). The NS proteins encode enzymatic functions necessary for RNA replication and evasion of host immune responses.
Overview of dengue pathology
In susceptible human hosts, DENV is thought to infect Langerhans cells at the site of mosquito inoculation, which then migrates through the lymphatics. There, the infection then spreads to other cells of hematopoietic lineages, including monocytes and macrophages, before further systemic dissemination [12–14]. Viremia becomes apparent 1–2 days prior to the onset of symptoms, peaks during days 1–2 of fever and declines over next 3–5 days until resolution of fever [9,11,15]. DENV infection stimulates both innate and acquired immunity. Innate immune response, such as complement and type I interferon (IFN), functions as the first line of defense against DENV [16,17]. Acquired immune response involves serotype-specific CD4+ and CD8+ T cells, which can result in lysis of DENV-infected cells and production of cytokines such as IFN-γ, TNF-α and lymphotoxin [9,18,19]. Antibody response to DENV is primarily targeted at prM, E and NS1 viral proteins [20–22]. Antibodies may neutralize DENV by blocking virus attachment to cells or by blocking viral fusion with cellular membranes [23,24]. Conversely, if antibody titers are below the threshold necessary for DENV neutralization, they may opsonize DENV and facilitate viral entry into cells that express Fcγ receptors more efficiently. The result is an increase in virus replication and higher risk of severe dengue, a phenomenon termed antibody-dependent enhancement (ADE) of infection [25–28].
• Clinical presentation
Approximately a quarter of dengue infections are symptomatic. In those who develop symptomatic infection, there is a wide spectrum of presentation and often with unpredictable clinical evolution and outcomes [15,29].
The symptomatic phase of dengue is summarized in the latest WHO guidelines and is divided into three phases: the febrile phase, critical phase and recovery phase [15]. After an incubation period of 4–10 days, the febrile phase begins abruptly and lasts for 2–7 days. It is characterized by acute fever and constitutional symptoms which could be debilitating (including chills, malaise, headache, arthralgia, myalgia, retro-orbital pain, anorexia, nausea, vomiting, lethargy and rash). Thrombocytopenia, leukopenia and variable rise in hematocrit (which indicates vascular leak) develop. Dehydration is commonly due to pyrexia, anorexia and vomiting [15,18].
The critical phase coincides with fever defervescence and viremia resolution, lasting approximately 24–48 h. There is associated capillary leakage, plasma volume loss and, if untreated or improperly managed, shock [7,15]. It is hypothesized that clinical complications are virus-driven immunological responses [30–37].
If the patient survives the critical phase, recovery phase begins after 48–72 h. Patient’s symptoms improve, and hemodynamic status stabilizes with reabsorption of extravasated fluid, along with rapid improvement in white cell and platelet counts. However, if excessive fluid therapy has been administered, patient may develop ascites and respiratory distress from massive pleural effusion, pulmonary edema or congestive heart failure [15,38].
• Rationale for dengue therapy
Currently, treatment for dengue is largely supportive. There is no licensed therapeutic drug. Several clinical trials of therapeutic interventions against acute dengue have been conducted and several more are in progress. These therapies target the virus, host immune responses or host factors required by DENV to complete replication cycle. Some clinical studies reported higher viremia levels (>tenfold) in patients with severe (dengue hemorrhagic fever [DHF]/dengue shock syndrome [DSS]) compared with nonsevere (dengue fever) during early infection [30,39–41]. This observed trend has led to the hypothesis that reducing viremia by antiviral therapy in the early phase of dengue infection may reduce severity of clinically apparent disease [30,42,43].
This review focuses on the clinical intervention that is widely and currently considered the gold standard in case management as well as those that have been tested in clinical trials as treatment for dengue. However, while development in dengue therapeutics is in progress, a number of practical challenges are already apparent. Patients may present to healthcare settings too late in their illness to benefit from antiviral therapy. Differentiating dengue from other febrile diseases is clinically difficult and diagnostic tests are usually lacking in resource-limited settings. Identifying patients at risk of severe disease and therefore most likely to benefit from antiviral treatment remains challenging. Furthermore, as viremia declines rapidly after illness onset, antiviral treatment must be fast acting. Dengue therapeutics development must therefore be coupled with improved diagnostic and prognostic tools in order to translate research into clinical practice.
• Supportive therapies during acute dengue
Fluid therapy
Sensible fluid resuscitation is of paramount importance in the management of patients with severe dengue, and a clinical algorithm has been published in detail in the latest WHO guidelines [15]. The goal is to prevent the complications of vascular leakage and hypovolemic shock.
Theoretically, colloids provide volume expansion over and above the actual fluid volume infused. Colloid molecules increase plasma oncotic pressure and promote retention of fluid in the intravascular compartment. The magnitude of this effect is determined by the average molecular weight of the colloid molecule and circulation retention time determines the duration of the effect [44,45]. For crystalloids, the plasma-volume-expanding capacity is related to sodium concentration. NaCl 0.9% (154 mM) in theory may perform better than Ringer’s lactate (131 mM). In addition, there are theoretical risks of worsening tissue acidosis and lactate accumulation when large volumes of Ringer’s lactate are infused [44,45]. However, Wills et al. compared Ringer’s lactate with two colloids and observed no significant advantage of colloid compared with lactate infusion in treating children with DSS [46]. In addition, the effects of colloid were transient, despite early rebound of hematocrit in children receiving colloids during initial resuscitation; there was no difference between the different fluid regimens in terms of outcome and severity of fluid overload 48–72 h after study infusion.
Besides the study by Wills et al., three other trials have been conducted to compare the different types of fluid resuscitation regimen of DSS. Table 1 summarizes all four such randomized controlled trials [44,46–48]. Taken collectively, there is no clear advantage to the use of colloids in terms of the overall outcome. However, colloids may be the preferred choice if blood pressure needs to be restored urgently (in those with pulse pressure <10 mmHg) as they have been shown to restore the cardiac index and hematocrit faster than crystalloids in patients with intractable shock [44,46–48]. Furthermore, the overall low (0–0.2%) mortality of the study population supports the importance of carefully titrated fluid therapy to maintain vital functions during the vascular leakage period without overfilling the intravascular space and meticulous supportive care for patients with DSS.
Blood products to reduce bleeding complications
Thrombocytopenia (platelet count below 150 × 109/l) is a hallmark of DENV infection and usually observed between days 3 and 8 following the onset of illness. Platelet count plummets in parallel with a rising hematocrit, indicative of progression to the critical phase of the disease. Platelet count reaches nadir during defervescence (days 3–6), which is then followed by gradual spontaneous recovery [15,18]. Minor bleeding (mucosal, petechiae) without hemodynamic instability is common and usually resolves spontaneously. In patients with severe thrombocytopenia (defined as ≤20 × 109/l), strict bed rest and avoidance of nonsteroidal anti-inflammatory drugs and intramuscular injections alone are usually sufficient to reduce the risk of severe bleeding [15].
Prophylactic platelet transfusion in patients without bleeding when platelet falls below 10–20 × 109/l is widely practiced in sepsis [49], but is not supported by evidence in dengue management. First, a clear inverse correlation between platelet count and bleeding risk is lacking [50–53]. Rather, the two risk factors of severe bleeding are prolonged shock and normal–low hematocrit at the diagnosis of shock [50]. A summary of platelet and fresh frozen plasma transfusion trials (Table 2) shows that current data do not support prophylactic platelet transfusion given the lack of sustainable and significant benefits [52,54,55]. Furthermore, the benefits of treatment are outweighed by significant risks including fluid overload, transfusion-associated lung injury, blood-borne infections and allergic reactions.
When major bleeding occurs, it usually arises from the gastrointestinal tract and/or vagina in adult females. Patients at risk of major bleeding include those who have: first, prolonged or refractory shock; second, renal or liver failure; third, persistent metabolic acidosis; fourth, taken nonsteroidal anti-inflammatory drugs or anticoagulants (e.g., heparin, warfarin) and fifth, preexisting peptic ulcer disease [15]. In the event of severe bleeding, timely transfusion of packed red cells, platelets and fresh frozen plasma may be lifesaving.
• Therapies targeting the host immune response
Corticosteroids
Corticosteroids have inhibitory effects on a broad range of immune responses mediated by T and B cells as well as native immune responses of phagocytes. High-dose corticosteroids are of benefit in many conditions with immune aberrancy, notably autoimmune diseases such as systemic lupus erythematous [56]. In a double-blind placebo-controlled trial, the use of corticosteroids during the early acute phase of dengue infection showed no effects on shock, plasma leakage or platelet count recovery in dengue patients. It also did not change the kinetics of dengue virological markers or plasma cytokine concentrations (Table 3) [57–63]. In summary, the use of steroids cannot be recommended [64].
Intravenous immunoglobulins
Intravenous immunoglobulins (IVIG) is an accepted treatment for idiopathic thrombocytopenia purpura (ITP). Thrombocytopenia in ITP is attributed to autoantibodies called platelet-associated IgG (PAIgG). PAIgG-coated platelets undergo accelerated clearance through Fcγ receptors expressed on mononuclear phagocytic cells. The mechanism of IVIG is probably via competitive inhibition of Fcγ receptors or ligation of inhibitory receptors [25,26,65].
A randomized, controlled study of 36 dengue patients was conducted to evaluate treatment with IVIG according to the dosage and frequency used for treatment of ITP for total of 4 days [66]. The study failed to show efficacy of IVIG in promoting platelet recovery.
• Mast cell inhibitors
Recent studies by St John et al. identified the role of Mast cell (MC) activation in the pathogenesis of dengue-vascular leakage and hemorrhage [67,68]. During DENV infection, activated MC was shown in a mouse model to release various proteases, particularly chymase and tryptase, into the serum that results in loss of vascular integrity. Consistent with the observation in mice, the authors also showed that serum chymase levels correlated with dengue severity in patients enrolled in a prospective study. The team then showed that MC-stabilizing compounds, including cromolyn, montelukast and ketotifen, reduced vascular leakage in wild-type mice model of DENV challenge despite small (but nonsignificant) increase in mice viremia [67]. Together, these data highlight the potential of MCs as therapeutic targets to limit DENV pathology. A proof-of-concept clinical trial to test the efficacy of ketotifen to reduce the degree of vascular leakage is currently in progress in Singapore [Tambyah PA, St John A; Pers. Comm.].
Target profile for a dengue therapeutic
There is still an unmet need for an effective antidengue drug that can shorten the duration of illness and prevent the development of severe complications. Ideal properties for a future dengue therapeutics are summarized in Table 4. The life cycle of DENV, drug targets and candidate drugs are summarized in Figure 1 and reviewed in detail elsewhere [69].
• Drugs targeting host factors required by DENV to complete its life cycle
Chloroquine
Chloroquine (CQ) was the first drug that underwent clinical trial with reduction of viral load as its primary end point [70]. CQ is a cheap and widely available lysosomotrophic 4-amino-quinoline derivative that is well established for the treatment of malaria and inflammatory disorders such as rheumatoid arthritis and systemic lupus erythematous. In vitro, CQ inhibits flaviviruses, retroviruses and coronaviruses by interfering with pH-dependent steps of viral replication. In DENV infection, CQ inhibits endosomal fusion and furin-dependent viral maturation, both of which are dependent on low-pH environment in the endosome and trans-Golgi network, respectively [70,71]. CQ also has immunomodulatory effects by suppressing release of TNF-α and IL-6, both of which may be responsible for the inflammatory complications of viral infections [72].
In a randomized, double-blind placebo-controlled trial of 307 Vietnamese adult patients hospitalized with laboratory-confirmed DENV infection, CQ-treated patients had longer duration of viremia [70]. There was also no apparent reduction in the development of DHF. Furthermore, CQ treatment was associated with a significant increase in the rate of vomiting. In a more recent small, randomized, double-blind study of 37 positive dengue patients, CQ treatment was shown to reduce the severity of pain symptoms but failed to reduce disease duration, the degree or days of fever [73].
Celgosivir
DENV assembly occurs in the endoplasmic reticulum (ER), where heterodimers of prM and E proteins localize to the luminal side of ER to form an immature particle. N-linked glycosylation of prM and E proteins is required for viral assembly and release of mature, infectious DENV particles [15,74]. DENV infection also induces ER stress, which activates the unfolded protein response machinery. The expression of UPR genes in turn activates various signaling pathways that induce apoptosis [75].
Celgosivir is an alkaloid castanospermine derived from the Moreton Bay Chestnut tree [75]. Following oral administration, it readily crosses cell membranes and is converted to Cast, which inhibits the catalytic activity of ER-resident enzymes, alpha-glucosidase I and II. Both enzymes catalyze the removal of terminal glucose residue attached to N-linked glycans of newly synthesized glycoproteins. Inhibition therefore is thought to result in misfolding of E, prM and NS1 proteins during virus replication. Celgosivir could also reduce cellular apoptosis due to virus-induced ER stress [75]. In vitro, celgosivir inhibited all four serotypes at submicromolar concentrations [76]. Celgosivir-treated mice showed reduced viremia, robust immune response and increased survival upon lethal DENV challenge, even when treatment was delayed by 48 h after infection [75]. Celgosivir was tested in Phase I and II trials as a possible treatment for HIV and hepatitis C infection and was found to be safe [75,76]. However, the drug was not further developed because it failed to demonstrate superiority over existing treatments.
A Phase Ib, randomized, double-blind, placebo-controlled, proof-of-concept, single-centered trial to evaluate the safety and efficacy of celgosivir as an antidengue drug (CELADEN) was conducted in Singapore [77]. It recruited 50 PCR-confirmed adult dengue patients within the first 2 days from illness onset. Individuals were randomized to receive celgosivir for 5 days or matched placebo. Results showed that celgosivir treatment did not significantly reduce viremia, fever or pain scores in patients with dengue. White blood cell and platelet counts as well as changes in hematocrit levels over the course of illness were not different between treated and placebo groups. Thus, the two proof-of-concept trials for inhibitors of host factors of DENV failed to show useful therapeutic efficacy.
Others (HMG-CoA-reductase inhibitors)
Inhibition of cholesterol synthesis may result in faulty viral particle assembly and protein glycosylation. 3-Hydroxy-3-methylglutaryl coenzyme A (HMG-CoA) reductase inhibitors, known as statins, have cholesterol-lowering effects and established roles in cardiovascular risk reduction [78]. They also exhibit anti-inflammatory and endothelial-stabilizing effects and possible antiviral effect targeting DENV virion assembly [79]. In an AG129 model of DENV-2 infection, lovastatin increased survival rate when administered either before or after infection [78]. However, it only reduced viral load if given before DENV-2 infection [78]. A randomized controlled trial to evaluate the effects of lovastatin on adult dengue patients is in progress in Vietnam [80].
• Drugs targeting dengue proteins
Various direct-acting antiviral agents (DAAs) have been developed that target specific dengue proteins. Interested readers can refer to these excellent reviews on the latest developments, chemical structures and mechanisms of actions of individual novel DAAs and therapeutic antibodies, which will not be discussed in detail here [81–83]. Instead, we have provided broad overviews of the development of these approaches to treating dengue.
Entry/fusion inhibitors
Entry of DENV into the host cell is mediated mainly by E protein, which contains three domains (DI, DII, DIII) [84]. In the mature DENV particle, E protein is organized into 90 homodimers with the fusion loop concealed inside the dimer interface. The first step of virus entry is the binding of viral E protein to several types of cellular receptors and/or attachment factors (e.g., DC-SIGN, heparin sulfate, mannose receptor, CD14, GRP78, laminin receptor and TAM proteins) [85]. Following binding, virus becomes internalized via clathrin-mediated endocytosis [85]. Inside the cell, the acidic pH environment induces conformational change and rearrangements of the E protein to expose and insert the fusion loop into the endosomal membrane, followed by membrane fusion pore formation to allow viral RNA to enter the cytoplasm. Membrane fusion inhibitors bind to various portions of the structural envelope and C proteins have been developed and recently reviewed [85]. Their future use awaits further optimization, animal and clinical studies [82].
Replication & transcription inhibitors
RNA-dependent RNA polymerase inhibitor (balapiravir)
NS5 is the largest and most conserved nonstructural protein in DENV as well as other flaviviruses. NS5 contains two enzymatic domains: first, the N-terminal methyltransferase (NS5-MTase) domain and second, the C-terminal RNA-dependent RNA polymerase (RdRP) domain (NS5-pol). Balapiravir is a product of a nucleoside analog (4’-azidocytine) called R1479, which was initially developed for the treatment of chronic HCV infection [86]. This drug inhibits viral NS5 protein, thereby reducing RdRP-dependent RNA synthesis. It is not licensed for use in HCV due to bone marrow suppression that arose with long-term treatment given with pegylated IFN and ribavirin [87]. Dengue and hepatitis C share similar RdRP architecture. A randomized, double-blind placebo-controlled trial was thus conducted to study the effect of a 5-day course of balapiravir prescribed during the first 48 h of illness onset in adult dengue patients [86]. Although the drug was well tolerated, it failed to reduce either viremia, NS1 antigenemia or fever clearance time. Plasma cytokine profile and whole blood transcriptional profile were not significantly different between treatment groups. In conclusion, this trial did not support balapiravir as a candidate drug.
MTase inhibitors
The NS5-MTase domain catalyzes RNA cap methylation at both the N7 position of the guanine cap and the 2’O position of the first nucleotide of the newly synthesized positive-strand RNA [88]. It can further methylate internal adenosine of the viral RNA genome at the ribose 2’-OH position. N7 methylation of RNA cap is essential for efficient translation, as mutations of MTase that abolish N7 methylation are lethal for flaviviral replication [88]. New compounds (e.g., ‘compound 10’) are being developed that selectively bind and inhibit MTase. At present, suitable compounds are still being screened for further testing [89].
Nucleoside analog (NITD008)
An adenosine analog NITD008 was developed that exhibits antiviral effect via inhibition of DENV RdRP and termination of RNA chain synthesis [90]. In vitro studies showed that NITD008 reduced DENV 1–4 replication. DENV-infected mice treated with NITD008 demonstrated reductions in viral load, proinflammatory cytokines and mortality. However, this drug also showed significant side effects during preclinical toxicology studies in mice, and therefore not further developed for clinical trials [90].
Helicase inhibitors
Helicase inhibitors work by inhibiting DENV NS3 unwinding activity during RNA replication [91]. Recently, a novel small-molecule helicase inhibitor (ST-610) was identified. This compound potently and selectively inhibited all four serotypes of DENV in vitro [92]. In mice, it was well tolerated and effective in reducing viremia. However, this drug has poor oral bioavailability and requires parenteral administration, hence limiting its use especially in resource-limited settings.
Protease inhibitors
The DENV NS3 is a serine protease in association with NS2B as a cofactor. During DENV replication, correct co- and post-translational processing of the polypeptide gives rise to the structural and nonstructural proteins and is therefore essential for virus replication [93]. These steps require multiple proteases derived from the host (e.g., furin) and virus (NS2B/NS3). Viral proteases are proven antiviral targets as demonstrated by the highly effective HIV-1 and HCV protease inhibitors [93]. DENV 1–4 NS3 protease shares 63–74% sequence similarity, suggesting that although it is plausible to develop effective protease inhibitors against all four DENV serotypes, the barrier to resistance could be low. Several potential protease inhibitors have been identified (including 166347, ARDP006, thyrothricin, compound 23i and compound 7n), but their development is still in its infancy and to date no drugs have been evaluated in vivo [82].
NS4B inhibitor
NS4B is a transmembrane protein without identified enzymatic activity but is required for the formation and anchorage of the active viral replication complex onto ER membranes [94]. NS4B also interferes with IFN-α/β signaling to promote virus propagation [95]. Several groups have identified compounds that inhibit DENV replication by targeting NS4B. NITD-618 was one of the compounds that was identified and demonstrated to be active against all four DENV serotypes [96]. However, NITD618 is highly lipophilic, which resulted in poor pharmacokinetic properties that hindered its testing in vivo [82]. Lycorine, a plant alkaloid, was another NS4B inhibitor with ability to inhibit WNV, YFV and DENV 1–2 replication [97,98]. Another compound, SDM25N, was shown to be active against DENV 2 in vitro [99]. The efficacy, safety and ultimate clinical utility of NS4B inhibitors await further clinical research.
Therapeutic antibodies
Therapeutic human serum polyclonal antibodies (IgG), prepared from pools of plasma obtained from multiple healthy blood donors, is assumed to contain wide array of antibodies that would be present in normal human serum and against common viral pathogens [100]. However, the disadvantage of polyclonal antibodies includes batch-to-batch variations, risk of blood-borne pathogens and multiple side effects especially allergic reactions [101]. Furthermore, batches must be screened to ensure neutralization activity against all four DENV serotypes [21]. Therapeutic monoclonal antibodies (mAbs), in contrast, can be produced in high quantities, specificity and consistency with significantly reduced adverse events associated with polyclonal IgGs [83].
Antibodies targeting the lateral ridge or A-strand of E protein EDIII can potently neutralize some but rarely all four serotypes [102]. An A-strand-specific mAb named 4E11 was redesigned through computational chemistry to neutralize all four serotypes in vitro and in mouse model of DENV challenge [103]. More recently, the team further developed this humanized antibody by using a combination of computational protein chemistry and X-ray crystallography to further improve this antibody for therapeutic application [104]. This antibody is currently undergoing preclinical development.
Alternatively, mAbs isolated from convalescent dengue patients that neutralize all DENVs with remarkable potency may also have therapeutic potential. Unlike mouse-derived antibodies, human mAbs mostly bind quaternary epitopes. de Alwis et al. identified a potent serotype-specific mAb that binds the hinge region between EDI and EDII [105]. Teoh et al. identified a potent serotype-specific mAb HM14c10 that binds a discontinuous epitope spanning adjacent surfaces of E-protein dimers on DENV-1 [106]. Fibriansah et al. showed that a highly potent mAb against DENV-3 binds the functionally important domains across DI–III proteins [107]. The same group also identified a DENV2-specific human mAb 2D22 that binds across E proteins and blocks the E-protein structural reorganization required for virus fusion [108]. Dejnirattisai et al. identified human mAbs that bind epitopes that bridge across two E proteins (called envelope dimer epitopes or EDE) [109]. Interestingly, these antibodies show pan-serotype neutralizing activity and could be useful therapeutically.
While therapeutic antibodies hold much promise, they have only been studied in vitro and in animal models. Molecular mechanisms that operate and potentially influence the efficacy outcome have been reviewed elsewhere [83]. While these may have to be considered when translating antibodies to therapeutic use, it would nonetheless be interesting to see the progress of this form of therapeutics into the clinical space as a treatment for an acute viral disease like dengue.
Conclusion
Despite the large number of candidate compounds that show antiviral effects against DENV in vitro, few have been evaluated in clinical trials. Although the optimal choice of fluids in acute dengue is still debatable, meticulous fluid therapy with close monitoring is the only established standard of care that ameliorates complications due to vascular leakage, shock and organ failure. Drug discovery is hindered by the lack of a reliable animal model of DENV infection and the long expensive process of new molecules discovery. It is hoped that efforts to sustain and perhaps even accelerate the translation of bench-based findings into therapeutic trials will continue in the coming years, especially since a fully preventative dengue vaccine remains challenging [112-114].
Future perspective
While an effective therapeutic against dengue remains elusive, the number of translational studies that have been carried out is encouraging. These efforts are long overdue against a disease that results in significant global health burden. As new studies are being developed, downstream studies necessary for clinical translation of any therapeutic should also be actively considered. Since the great majority of patients with dengue infection recover without progression to DHF/DSS, studies to demonstrate clinical benefits of therapy to prevent severe disease would therefore require recruitment of a very large number of patients. In this context, perhaps there is an urgent need to revisit human challenge studies that could serve as proof-of-concept clinical trials to complement therapeutic development. Such trials can provide clear and definitive efficacy signals and guide the design of larger trials. Such an approach would prevent prolonged and expensive clinical trials that often reach dead ends due to insufficiently robust efficacy. Proposition for human challenge studies has been discussed elsewhere [110,111].
Study (year) | Design | Setting | Patients | Participants (n) | Fluid | Intervention | Conclusion | Ref. |
---|---|---|---|---|---|---|---|---|
Dung et al. (1999) | Double-blind, randomized, controlled trial | Vietnam | Pediatrics ICU patients aged 5–15 years with DSS | 50 | NaCl 0.9%; dextran 7; 3% gelatin; Ringer’s lactate | Study fluid was administered for the first 72 h | Colloids may be superior to crystalloids, with more rapid restoration of hematocrit and cardiovascular stability | [47] |
Ngo et al. (2001) | Double-blind, randomized trial | Vietnam | Pediatric ICU patients (aged 1–15 years) with DSS without severe hemorrhage | 222 | Dextran 70; 3% gelatin; Ringer’s lactate; NaCl 0.9% | Study fluid was administered for the first 1 h, followed by Ringer’s lactate (WHO guideline*) | No clear advantage to using any of the fluids in cardiovascular recovery time. Colloids were more likely to benefit patients severe shock | [44] |
Wills et al. (2005) | Double-blind, randomized trial | Vietnam | Pediatric ICU patients (aged 2–15 years) with DSS | 383 with moderately severe DSS; 129 with severe DSS | Moderately severe DSS group received crystalloid (Ringer’s lactate) vs colloid (6% dextran 70 or 6% hydroxylethyl starch). Severe DSS group received dextran 70 vs starch | Study fluid was administered for the first 2 h, followed by Ringer’s lactate*. Patients with persistent shock after administration of study fluid were given rescue colloids | Requirement for rescue colloid was similar for the different regimens. Significantly, more recipients in the dextran group had coagulopathy and fluid overload than starch. Colloids performed similarly in severe DSS. Ringer’s lactate should be used in children with moderately severe DSS | [46] |
Kalayanarooj (2008) | Randomized, single-blinded trial | Thailand | Pediatric DHF patients (aged 8.6 ± 3.9 years) who initially received crystalloid, but failed to improve or developed fluid overload | 104 | Colloids – 10% hydroxyethyl starch vs 10% dextran 40 | Doses of study colloids were given in boluses when indicated at clinician’s discretion | Both colloids were equally effective with no difference in complications with regard to fluid overload, renal functions and blood coagulation | [48] |
Study (year) | Design | Setting | Patients | Participants (n) | Treatment | Conclusion | Favor transfusion? | Ref. |
---|---|---|---|---|---|---|---|---|
Sellahewa et al. (2008) | Randomized, double-blind-controlled trial | Sri Lanka | Dengue adult patients with platelet count <40,000/mm3 without bleeding | 109 | Prophylactic FFP | FFP may contribute to an increased platelet count in the first 12 h, but no difference was observed at 24–48 h | No | [54] |
Assir et al. (2013) | Randomized, nonblinded trial | Pakistan | Adult dengue patients with platelet count <30,000/µl without bleeding or with mild bleeding | 87 | Prophylactic platelets | No benefit in the prevention or resolution of bleeding. 7% developed severe side effect | No | [55] |
Lye et al. (2009) | Nonrandomized, retrospective cohort study | Singapore | Adult patients with severe thrombocytopenia (<20 × 103/µl) without clinical bleeding | 266 | Prophylactic platelets | No benefit | No | [52] |
Study (year) | Design | Setting | Patients | Participants (n) | Drug | Conclusion | Favor steroids? | Ref. |
---|---|---|---|---|---|---|---|---|
Pongpanich et al. (1973) | Nonblinded, randomized | Thailand | Children with dengue shock | 26 | IV hydrocortisone | No difference in duration of shock or need for fluid replacement | No | [57] |
Min et al. (1975) | Randomized, double-blinded | Thailand | Children with dengue shock | 98 | IV hydrocortisone for 3 days | Favors steroids in children >8 years. Case fatality 44% (22 of 50) in nonsteroid group, and 18.75% (9 of 48) in steroid group | Yes | [58] |
Sumarmo et al. (1982) | Randomized, nonblinded | Indonesia | Children <10 years with dengue shock | 97 | IV hydrocortisone × 1 dose | No difference in duration of shock or need for fluid requirement. No difference in mortality | No | [59] |
Tassniyom et al. (1993) | Randomized, double-blinded | Thailand | Children <15 years with dengue shock | 63 | IV methylprednisolone × 1 dose | No difference in mortality | No | [60] |
Kularatne et al. (2009) | Randomized, placebo-controlled, double-blinded | Sri Lanka | Dengue patients aged 12–65 years with platelets <50 × 109/l | 200 | IV dexamethasone for 24 h | Ineffective in increasing platelet count | No | [61] |
Tam et al. (2012) | Randomized, placebo-controlled, double-blind | Vietnam | Dengue patients aged 5–20 years ≤72 h | 255 | Oral prednisolone for 3 days | Steroids not associated with prolonged viremia | (Underpowered) | [62] |
Shashidhara et al. (2013) | Randomized, controlled, nonblinded | India | Adults with dengue aged >18 years | 61 | IV dexamethasone for 4 days | Ineffective in increasing platelet count | No | [63] |
Drug parameters | Ideal properties |
---|---|
Spectrum | Effective against all four DENV serotypes |
Clinical outcome | Rapid resolution of symptoms, reduced severity |
Safety | Well tolerated, minimal toxicity and does not require lab monitoring |
Bioavailability | Fast acting, high volume of distribution |
Pharmacokinetic | Sufficient half-life to enable infrequent (e.g., once daily) dosing to promote compliance |
Route of administration | Oral rather than parenteral |
Interactions | Minimal with common drugs |
Target population | Adults, children, infants, pregnant women, patients with co-morbidities (renal, hepatic) |
Others | Long shelf-life, stable without refrigeration to facilitate rural distribution |
Cost | Affordable in dengue-endemic countries |
EXECUTIVE SUMMARY
Spectrum of dengue infection
Dengue is an acute, systemic viral infection caused by one of four dengue serotypes in the family Flaviviridae, transmitted by Aedes mosquitoes.
Dengue occurs primarily in the tropics.
Dengue presents with wide clinical spectrum, ranging from subclinical infection, or may cause mild dengue fever (DF), to severe and even fatal dengue hemorrhagic fever (DHF) or dengue shock syndrome (DSS).
Death is usually primarily by plasma leakage, leading to shock, organ failure and hemorrhage.
Three phases of disease exist – febrile phase, critical phase and recovery phase.
There is no licensed drug or highly effective vaccine available at present.
Clinical assessment (history, physical examination and vital signs) and laboratory tests (complete blood count and hematocrit) are required to assess disease phase and severity.
Dengue treatment: supportive therapy during acute infection
Judicious therapy is currently the cornerstone of managing acute dengue to prevent complications due to plasma leakage, shock, organ failure and bleeding. However, excessive fluid can result in fluid overload.
There is no clear advantage to the use of colloids over crystalloids in outcome. Colloids may be preferred in severe (pulse pressure ≤10 mmHg) or refractory shock to quickly improve hemodynamic status.
Blood product transfusion is indicated in patients with major hemorrhage.
Thrombocytopenia is common in dengue during the febrile phase, but overt bleeding is uncommon. Prophylactic platelet or fresh frozen plasma transfusion for severe thrombocytopenia in hemodynamically stable patients is not beneficial and not recommended.
Dengue treatment: drugs targeting host immune response
There is no evidence to support the use of intravenous immunoglobulins or corticosteroids in dengue and are not recommended.
Mast cells may be implicated in severe dengue via release of chymase and tryptase into the serum, causing vascular leakage. So far, macrophage-stabilizing compounds have showed promising results in improving outcomes in mouse models. Its therapeutic use in humans awaits clinical trials.
Drugs targeting host factors required by dengue virus to complete its life cycle
Celgosivir, an alpha-glucosidase inhibitor, effectively inhibits dengue virus (DENV) 1–4 in vitro. In Phase Ib clinical trial, it failed to demonstrate viral load reduction and clinical benefit.
Chloroquine, a lysosomotriphic 4-amino-quinoline derivative, inhibits dengue through pH-dependent steps of viral replication cycle and has ability to suppress cytokine-mediated inflammatory responses. No clinical benefit was observed based on two randomized, double-blind studies of dengue patients.
Cholesterol-lowering drugs (statins) may exhibit anti-inflammatory and endothelial-stabilizing effects and possible antiviral effect targeting DENV assembly. Lovastatin demonstrated survival benefits in mouse model of DENV infection. Its use in humans awaits clinical trials.
Drugs targeting dengue proteins or direct-acting antiviral agents
Drugs that target critical stages of dengue replication cycle (entry, fusion, translation, genome replication, protein synthesis and egress) have been extensively studied. Most of these drugs have not been studied in animal models or humans.
Balapiravir acts via inhibition of NS5/RdRP-dependent RNA synthesis. It is the only direct-acting antiviral agent (DAA) that entered clinical trial. However, treatment with balapiravir failed to reduce NS1 antigenemia, viral load and fever clearance time in dengue patients.
NS4B inhibitors act via inhibition of viral replication and prevents viral downregulation of interferon response.
Therapeutic mAbs that potently neutralize DENV by binding to the quaternary structures of envelope proteins without antibody-dependent enhancement could potentially be used against homologous serotypes.
To date, no licensed dengue DAAs are currently available.
Financial & competing interests disclosure
The authors have no relevant affiliations or financial involvement with any organization or entity with a financial interest in or financial conflict with the subject matter or materials discussed in the manuscript. This includes employment, consultancies, honoraria, stock ownership or options, expert testimony, grants or patents received or pending, or royalties.
No writing assistance was utilized in the production of this manuscript.
Papers of special note have been highlighted as: • of interest; •• of considerable interest
References
- 1 The global distribution and burden of dengue. Nature 496(7446), 504–507 (2013).• Provides review on dengue epidemiology and global distribution.
- 2 Evaluating diagnostics. Nature 8(12), S7–S16 (2010).• Provides review on global epidemiology and prevention strategies.
- 3 CDC. http://www.cdc.gov/dengue/epidemiology
- 4 . Dengue, urbanization and globalization: the unholy trinity of the 21st century. Trop. Med. Health 39(Supplement), S3–S11 (2011).
- 5 . Epidemic dengue/dengue hemorrhagic fever as a public health, social and economic problem in the 21st century. Trends Microbiol. 10(2), 100–103 (2002).
- 6 . The zoonotic flaviviruses of southern, south-eastern and eastern Asia, and Australasia: the potential for emergent viruses. Zoonoses Public Health 56(6–7), 338–356 (2009).
- 7 World Health Organization. Dengue, Dengue Haemorrhagic Fever and Dengue Shock Syndrome in the Context of the Integrated Management of Childhood Illness. WHO, Geneva, Switzerland, 1–40 (2005).
- 8 Health economics of dengue: a systematic literature review and expert panel’s assessment. Am. J. Trop. Med. Hyg. 84(3), 473–488 (2011).
- 9 . Immunity to dengue virus: a tale of original antigenic sin and tropical cytokine storms. Nature 11(8), 532–543 (2011).
- 10 . Discovery of fifth serotype of dengue virus (DENV-5): a new public health dilemma in dengue control. Med. J. Armed Forces India 71(1), 67–70 (2015).
- 11 . Seminar: dengue. Lancet 385(9966), 453–465 (2015). •• Provides excellent review on dengue clinical presentations, pathogenesis and management.
- 12 . Cells in dengue virus infection in vivo. Adv. Virol. 2010(5), 164878 (2010).
- 13 . Dendritic cells in dengue virus infection: targets of virus replication and mediators of immunity. Front. Immunol. 5, 647 (2014).
- 14 . Trafficking and replication patterns reveal splenic macrophages as major targets of dengue virus in mice. J. Virol. 86(22), 12138–12147 (2012).
- 15 World Health Organization. DENGUE: Guidelines for Diagnosis, Treatment, Prevention and Control (New Edition). WHO, Geneva, Switzerland, 1–160 (2009). •• Provides current clinical guideline on diagnosis and management of acute dengue infection.
- 16 . Innate immunity to dengue virus infection and subversion of antiviral responses. J. Mol. Biol. 426(6), 1148–1160 (2014).• Reviews on innate response against dengue and subversion of antiviral response.
- 17 . Innate immunity evasion by dengue virus. Viruses 4(12), 397–413 (2012).
- 18 . Pathogenesis of dengue virus infection. UpToDate, Waltham, MA, USA.• Provides review on dengue clinical presentation and pathogenesis.
- 19 . Bystander target cell lysis and cytokine production by dengue virus-specific human CD4(+) cytotoxic T-lymphocyte clones. J. Virol. 73(5), 3623–3629 (1999).
- 20 In-depth analysis of the antibody response of individuals exposed to primary dengue virus infection. PLoS Negl. Trop. Dis. 5(6), e1188 (2011).
- 21 Cross-reacting antibodies enhance dengue virus infection in humans. Science 328(5979), 745–748 (2010).
- 22 The human immune response to dengue virus is dominated by highly cross-reactive antibodies endowed with neutralizing and enhancing activity. Cell Host Microbe 8(3), 271–283 (2010).
- 23 . Antibody response to dengue virus. Microbes Infect. 16 (9), 711–720 (2014).
- 24 . The human antibody response to dengue virus infection. Viruses 3(12), 2374–2395 (2011).
- 25 . Vaccine development as a means to control dengue virus pathogenesis: do we know enough? Ann. Rev. Virol. 1(1), 375–398 (2014).• Reviews on dengue vaccine development.
- 26 Ligation of Fc gamma receptor IIB inhibits antibody-dependent enhancement of dengue virus infection. Proc. Natl Acad. Sci. USA 108(30), 12479–12484 (2011).
- 27 . Secondary infection as a risk factor for dengue hemorrhagic fever/dengue shock syndrome: an historical perspective and role of antibody-dependent enhancement of infection. Arch. Virol. 158(7), 1445–1459 (2013).
- 28 Influence of the dengue serotype, previous dengue infection, and plasma viral load on clinical presentation and outcome during a dengue-2 and dengue-4 co-epidemic. Am. J. Trop. Med. Hyg. 78(6), 990–998 (2008).
- 29 Early dengue infection and outcome study (EDEN) – study design and preliminary findings. Ann. Acad. Med. Singap. 35(11), 783 (2006).
- 30 Differing influences of virus burden and immune activation on disease severity in secondary dengue-3 virus infections. J. Infect. Dis. 185(9), 1213–1221 (2002).
- 31 . Cellular and cytokine correlates of severe dengue infection. PLoS ONE 7(11), e50387 (2012).
- 32 Cytokine expression profile of dengue patients at different phases of illness. PLoS ONE 7(12), e52215 (2012).
- 33 Host gene expression profiling of dengue virus infection in cell lines and patients. PLoS Negl. Trop. Dis. 1(2), e86 (2007).
- 34 Characterization of early host responses in adults with dengue disease. BMC Infect. Dis. 11(1), 209 (2011).
- 35 Sequential waves of gene expression in patients with clinically defined dengue illnesses reveal subtle disease phases and predict disease severity. PLoS Negl. Trop. Dis. 7(7), e2298 (2013).
- 36 Genome-wide expression profiling deciphers host responses altered during dengue shock syndrome and reveals the role of innate immunity in severe dengue. PLoS ONE 5(7), e11671 (2010).
- 37 . Differences in global gene expression in peripheral blood mononuclear cells indicate a significant role of the innate responses in progression of dengue fever but not dengue hemorrhagic fever. J. Infect. Dis. 197(10), 1459–1467 (2008).
- 38 . Cardiovascular manifestations of the emerging dengue pandemic. Nat. Rev. Cardiol. 11(6), 335–345 (2014).
- 39 High levels of plasma dengue viral load during defervescence in patients with dengue hemorrhagic fever: implications for pathogenesis. Virology 305(2), 330–338 (2003).
- 40 Dengue and dengue hemorrhagic fever among adults: clinical outcomes related to viremia, serotypes, and antibody response. J. Infect. Dis. 197(6), 817–824 (2008).
- 41 Slower rates of clearance of viral load and virus-containing immune complexes in patients with dengue hemorrhagic fever. Clin. Infect. Dis. 43(8), 1023–1030 (2006).
- 42 Dengue viremia titer, antibody response pattern, and virus serotype correlate with disease severity. J. Infect. Dis. 181(1), 2–9 (2000).
- 43 . Prospective study of the duration and magnitude of viraemia in children hospitalised during the 1996–1997 dengue-2 outbreak in French Polynesia. J. Med. Virol. 60(4), 432–438 (2000).
- 44 Acute management of dengue shock syndrome: a randomized double-blind comparison of 4 intravenous fluid regimens in the first hour. Clin. Infect. Dis. 32(2), 204–213 (2001).
- 45 . Pharmacologic and clinical considerations in selecting crystalloid, colloidal, and oxygen-carrying resuscitation fluids, Part 1. Clin. Pharm. 12(5), 335–346 (1993).
- 46 Comparison of three fluid solutions for resuscitation in dengue shock syndrome. N. Engl. J. Med. 353(9), 877–889 (2005).
- 47 Fluid replacement in dengue shock syndrome: a randomized, double-blind comparison of four intravenous-fluid regimens. Clin. Infect. Dis. 29(4), 787–794 (1999).
- 48 . Choice of colloidal solutions in dengue hemorrhagic fever patients. J. Med. Assoc. Thai. 91(Suppl. 3), S97–S103 (2008).
- 49 Surviving sepsis campaign. Crit. Care Med. 41(2), 580–637 (2013).
- 50 . Risk factors for hemorrhage in severe dengue infections. J. Pediatr. 140(5), 629–631 (2002).
- 51 Confirmed adult dengue deaths in Singapore: 5 year multi-center retrospective study. BMC Infect. Dis. 11, 123 (2011).
- 52 . Lack of efficacy of prophylactic platelet transfusion for severe thrombocytopenia in adults with acute uncomplicated dengue infection. Clin. Infect. Dis. 48(9), 1262–1265 (2009).
- 53 Mechanisms of hemorrhage in dengue without circulatory collapse. Am. J. Trop. Med. Hyg. 65(6), 840–847 (2001).
- 54 . Is fresh frozen plasma effective for thrombocytopenia in adults with dengue fever? A prospective randomised double blind controlled study. Ceylon Med. J. 53(2), 36–40 (2008).
- 55 Effectiveness of platelet transfusion in dengue fever: a randomized controlled trial. Transfus. Med. Hemother. 40(5), 362–368 (2013).
- 56 American College of Rheumatology guidelines for screening, treatment, and management of lupus nephritis. Arthritis Care Res. 64(6), 797–808 (2012).
- 57 . Studies on dengue hemorrhagic fever. clinical study: an evaluation of steroids as a treatment. J. Med. Assoc. Thai. 56(1), 6–14 (1973).
- 58 . Hydrocortisone in the management of dengue shock syndrome. Southeast Asian J. Trop. Med. Public Health 6(4), 573–579 (1975).
- 59 . Failure of hydrocortisone to affect outcome in dengue shock syndrome. Pediatrics 69(1), 45–49 (1982).
- 60 . Failure of high-dose methylprednisolone in established dengue shock syndrome: a placebo-controlled, double-blind study. Pediatrics 92(1), 111–115 (1993).
- 61 Efficacy of low dose dexamethasone in severe thrombocytopenia caused by dengue fever: a placebo controlled study. Postgrad. Med. J. 85(1008), 525–529 (2009).
- 62 Effects of short-course oral corticosteroid therapy in early dengue infection in Vietnamese patients: a randomized, placebo-controlled trial. Clin. Infect. Dis. 55(9), 1216–1224 (2012).
- 63 . Effect of high dose of steroid on platelet count in acute stage of dengue fever with thrombocytopenia. J. Clin. Diagn. Res. 7(7), 1397–1400 (2013).
- 64 . Corticosteroids for treating dengue shock syndrome. Cochrane Database Syst. Rev. 3, CD003488 (2006).
- 65 . Anti-inflammatory activity of IVIG mediated through the inhibitory Fc receptor. Science 291(5503), 484–486 (2001).
- 66 Lack of efficacy of high-dose intravenous immunoglobulin treatment of severe thrombocytopenia in patients with secondary dengue virus infection. Am. J. Trop. Med. Hyg. 77(6), 1135–1138 (2007).
- 67 . Contributions of mast cells and vasoactive products, leukotrienes and chymase, to dengue virus-induced vascular leakage. eLife 2, e00481 (2013).
- 68 Immune surveillance by mast cells during dengue infection promotes natural killer (NK) and NKT-cell recruitment and viral clearance. Proc. Natl Acad. Sci. USA 108(22), 9190–9195 (2011).
- 69 . Coupling of replication and assembly in flaviviruses. Curr. Opin. Virol. 9, 134–142 (2014).
- 70 A randomized controlled trial of chloroquine for the treatment of dengue in Vietnamese adults. PLoS Negl. Trop. Dis. 4(8), e785 (2010).
- 71 . Structure of the dengue virus envelope protein after membrane fusion. Nature 427(6972), 313–319 (2004).
- 72 . Effects of chloroquine on viral infections: an old drug against today’s diseases? Lancet Infect. Dis. 3(11), 722–727 (2003).
- 73 . Chloroquine use improves dengue-related symptoms. Mem. Inst. Oswaldo Cruz. 108(5), 596–599 (2013).
- 74 . Alpha-glucosidase inhibitors reduce dengue virus production by affecting the initial steps of virion morphogenesis in the endoplasmic reticulum. J. Virol. 74(1), 564–572 (2000).
- 75 Antiviral research. Antiviral Res. 92(3), 453–460 (2011).
- 76 . Celgosivir, an alpha-glucosidase I inhibitor for the potential treatment of HCV infection. Curr. Opin. Investig. Drugs 10(8), 860–870 (2009).
- 77 Efficacy and safety of celgosivir in patients with dengue fever (CELADEN): a Phase 1b, randomised, double-blind, placebo-controlled, proof-of-concept trial. Lancet Infect. Dis. 14(8), 706–715 (2014).
- 78 . Lovastatin delays infection and increases survival rates in AG129 mice infected with dengue virus serotype 2. PLoS ONE 9(2), e87412 (2014).
- 79 . A new role for statins in sepsis. Crit. Care. 17(1), 105 (2012).
- 80 Lovastatin for adult patients with dengue: protocol for a randomised controlled trial. Trials 13(1), 203 (2012).
- 81 Dengue therapeutics, chemoprophylaxis, and allied tools: state of the art and future directions. PLoS Negl. Trop. Dis. 8(8), e3025 (2014).•• Provides review on antidengue drug development.
- 82 Ten years of dengue drug discovery: progress and prospects. Antiviral Res. 100(2), 500–519 (2013).•• Provides a detailed review on antidengue drug development.
- 83 . Therapeutic antibodies as a treatment option for dengue fever. Expert Rev. Anti-Infect. Ther. 11(11), 1147–1157 (2013).• Provides a detailed review on therapeutic antibodies as dengue treatment and prophylaxis.
- 84 . A toggle switch controls the low pH-triggered rearrangement and maturation of the dengue virus envelope proteins. Nat. Commun. 5, 3877 (2014).
- 85 . Progress in the identification of dengue virus entry/fusion inhibitors. BioMed. Res. Int. 2014, 825039 (2014). • Provides review on dengue fusion/entry inhibitors in development.
- 86 A randomized, double-blind placebo controlled trial of balapiravir, a polymerase inhibitor, in adult dengue patients. J. Infect. Dis. 207(9), 1442–1450 (2013).
- 87 Balapiravir plus peginterferon alfa-2a (40KD)/ribavirin in a randomized trial of hepatitis C genotype 1 patients. Ann. Hepatol. 11(1), 15–31 (2012).
- 88 . Flavivirus methyltransferase: a novel antiviral target. Antiviral Res. 80(1), 1–10 (2008).
- 89 Small molecule inhibitors that selectively block dengue virus methyltransferase. J. Biol. Chem. 286(8), 6233–6240 (2011).
- 90 An adenosine nucleoside inhibitor of dengue virus. Proc. Natl Acad. Sci. USA 106(48), 20435–20439 (2009).
- 91 The RNA helicase and nucleotide triphosphatase activities of the bovine viral diarrhea virus NS3 protein are essential for viral replication. J. Virol. 74(4), 1794–1800 (2000).
- 92 Novel benzoxazole inhibitor of dengue virus replication that targets the NS3 helicase. Antimicrob. Agents Chemother. 57(4), 1902–1912 (2013).
- 93 . Dengue protease activity: the structural integrity and interaction of NS2B with NS3 protease and its potential as a drug target. Biosci. Rep. 31(5), 399–409 (2011).
- 94 Dimerization of flavivirus NS4B protein. J. Virol. 88(6), 3379–3391 (2014).
- 95 . Innate immunity to dengue virus infection and subversion of antiviral responses. J. Mol. Biol. 426(6), 1148–1160 (2014).
- 96 Inhibition of dengue virus by targeting viral NS4B protein. J. Virol. 85(21), 11183–11195 (2011).
- 97 . Subcellular localization and membrane topology of the dengue virus type 2 non-structural protein 4B. J. Biol. Chem. 281(13), 8854–8863 (2006).
- 98 A single-amino acid substitution in West Nile virus 2K peptide between NS4A and NS4B confers resistance to lycorine, a flavivirus inhibitor. Virology 384(1), 242–252 (2009).
- 99 Identification of a new dengue virus inhibitor that targets the viral NS4B protein and restricts genomic RNA replication. Antiviral Res. 99(2), 165–171 (2013).
- 100 . In vitro antiviral and antibacterial activity of commercial intravenous immunoglobulin preparations – a potential role for adjuvant intravenous immunoglobulin therapy in infectious diseases. Transfus. Med. 12(2), 133–139 (2002).
- 101 . Intravenous immunoglobulin: adverse effects and safe administration. Clin. Rev. Allergy Immunol. 29(3), 173–184 (2005).
- 102 Binding of a neutralizing antibody to dengue virus alters the arrangement of surface glycoproteins. Nat. Struct. Mol. Biol. 15(3), 312–317 (2008).
- 103 Redesign of a cross-reactive antibody to dengue virus with broad-spectrum activity and increased in vivo potency. Proc. Natl Acad. Sci. USA 110(17), E1555–E1564 (2013).
- 104 Structure-guided design of an anti-dengue antibody directed to a non-immunodominant epitope. Cell 162(3), 493–504 (2015).
- 105 Identification of human neutralizing antibodies that bind to complex epitopes on dengue virions. Proc. Natl Acad. Sci. USA 109(19), 7439–7444 (2012).
- 106 The structural basis for serotype-specific neutralization of dengue virus by a human antibody. Sci. Transl. Med. 4(139), 139ra83–139ra83 (2012).
- 107 A highly potent human antibody neutralizes dengue virus serotype 3 by binding across three surface proteins. Nat. Commun. 6, 1–10 (2015).
- 108 Dengue virus. Cryo-EM structure of an antibody that neutralizes dengue virus type 2 by locking E protein dimers. Science 349(6243), 88–91 (2015).
- 109 A new class of highly potent, broadly neutralizing antibodies isolated from viremic patients infected with dengue virus. Nat. Immunol. 16(2), 170–177 (2015).
- 110 . The human dengue challenge experience at the Walter Reed Army Institute of Research. J. Infect. Dis. 209(Suppl. 2), S49–S55 (2014).
- 111 Evaluation of dengue virus strains for human challenge studies. Vaccine 32(13), 1488–1494 (2014).
- 112 Protective efficacy of the recombinant, live-attenuated, CYD tetravalent dengue vaccine in Thai schoolchildren: a randomised, controlled Phase 2b trial. Lancet 380(9853), 1559–1567 (2012).
- 113 . Preventing dengue – is the possibility now a reality? N. Engl. J. Med. 372(2), 172–173 (2015).
- 114 Efficacy of a tetravalent dengue vaccine in children in Latin America. N. Engl. J. Med. 372(2), 113–123 (2015).